In Situ Visualizing Carboxylesterase Activity in Type 2 Diabetes Mellitus Using an Activatable Endoplasmic Reticulum Targetable Proximity Labeling Far-Red Fluorescent Probe
Ningge Xu【胥宁格】,Dandan Tang【唐丹丹】,Heng Liu*【刘恒】,Mengyue Liu【刘梦月】,Zheng Wen【文正】,Tongmeng Jiang【蒋童蒙】*, and Fabiao Yu【于法标】*
Cite this: Anal. Chem. 2024, 96, 26, 10724–10731
Publication Date:June 19, 2024
https://doi.org/10.1021/acs.analchem.4c01721
1Department of Radiotherapy, The First Affiliated Hospital of Hainan Medical University, Hainan Medical University, Haikou, 570102, China
2Key Laboratory of Hainan Trauma and Disaster Rescue, Key Laboratory of Emergency and Trauma, Ministry of Education, Engineering Research Center for Hainan Bio-Smart Materials and Bio-Medical Devices, College of Emergency and Trauma, Hainan Medical University, Haikou 571199, China
羧酸酯酶(CE)是一种在生物体中普遍存在的酶,它参与多种关键的生理和病理过程。肝脏中CE水平的变化可能作为II型糖尿病(T2DM)的重要预兆。近期,海南医科大学于法标教授课题组报道了II型糖尿病中羧酸酯酶活性检测,相关成果以“In Situ Visualizing Carboxylesterase Activity in Type 2 Diabetes Mellitus Using an Activatable Endoplasmic Reticulum Targetable Proximity Labeling Far-Red Fluorescent Probe”为题发表在国际化学权威杂志Analytical Chemistry上(DOI: 10.1021/acs.analchem.4c01721)。
我们开发出了一种创新的远红荧光探针DCI2F-Ac,它基于二氰基异佛尔酮(DCI)设计,具备内质网靶向的邻近标记功能,用于实时、准确地监测和成像CE的活性。DCI2F-Ac具有卓越的安全性,表现为极低的细胞毒性和生物毒性,同时对CE显示出极高的选择性和敏感性。与传统的CE探针相比,DCI2F-Ac的独特之处在于它能够直接共价锚定到CE上,显著减少了因扩散导致的原位荧光信号损失,提高了测量的精确性。借助“开启-关闭”荧光信号模式,DCI2F-Ac不仅能够有效区分不同的细胞系,还能精确筛选CE抑制剂。在内质网(ER)应激的研究中,我们发现毒胡萝卜素(Tg)能够显著上调CE水平,而衣霉素(Tm)则不会引发类似效应,这一发现与ER的钙稳态紧密相关。特别值得注意的是,DCI2F-Ac在T2DM患者的肝脏样本中能够有效检测到CE活性的下降,并且我们可以通过追踪CE水平的变化来评估二甲双胍、阿卡波糖以及两者联合用药的治疗效果。实验结果显示,二甲双胍与阿卡波糖的联合使用能够显著恢复CE水平至接近正常范围,展现出最佳的抗糖尿病效果。因此,DCI2F-Ac探针不仅为肝脏代谢紊乱的研究提供了新的视角,也为药物疗效评估提供了有力的工具,为探索CE在相关疾病中的潜力开辟了新途径。海南医科大学功能材料与分子影像技术研究团队硕士生胥宁格和唐丹丹为论文共同第一作者,刘恒研究员和于法标教授为共同通讯作者。本研究受到国家自然科学基金和海南省重点研发计划等项目资助。
Abstract

Carboxylesterase (CE), an enzyme widely present in organisms, is involved in various physiological and pathological processes. Changes in the levels of CEs in the liver may predict the presence of type 2 diabetes mellitus (T2DM). Here, a novel dicyanoisophorone (DCI)-based proximity-labeled far-red fluorescent probe DCI2F-Ac with endoplasmic reticulum targeting was proposed for real-time monitoring and imaging of the CEs activity. DCI2F-Ac featured very low cytotoxicity and biotoxicity and was highly selective and sensitive for CEs. Compared with traditional CEs probes, DCI2F-Ac was covalently anchored directly to CEs, thus effectively reducing the loss of in situ fluorescent signals due to diffusion. Through the “on–off” fluorescence signal readout, DCI2F-Ac was able to distinguish cell lines and screen for CEs inhibitors. In terms of endoplasmic reticulum (ER) stress, it was found that thapsigargin (Tg) induced upregulation of CEs levels but not tunicamycin (Tm), which was related to the calcium homeostasis of the ER. DCI2F-Ac could efficiently detect downregulated CEs in the livers of T2DM, and the therapeutic efficacy of metformin, acarbose, and a combination of these two drugs was assessed by tracking the fluctuation of CEs levels. The results showed that combining metformin and acarbose could restore CEs levels to near-normal levels with the best antidiabetic effect. Thus, the DCI2F-Ac probe provides a great opportunity to explore the untapped potential of CEs in liver metabolic disorders and drug efficacy assessment.

Scheme 1. (a) Schematic illustration of dual sensing and labeling NIRF probe DCI2F-OTf for ONOO-. (b) Synthesis of NIRF probe DCI2F-OTf.

Figure 1. Fluorescence response of DCI2F-OTf (10 μM) to ONOO- excited at 490 nm. (a) Visualization of the fluorescence changes of DCI2F-OTf during titration with increasing ONOO- (0 - 35 μM) concentrations. (b) Linearity of the fluorescence intensity value of DCI2F-OTf at 652 nm with varied concentrations of ONOO- (5 - 35 μM). (c) Time-dependent fluorescence response of DCI2F-OTf before and after the addition of ONOO- (70 μM) at 37 °C recorded every 5 min. (d) The fluorescence intensities at 652 nm of DCI2F-OTf in the absence or presence of ONOO- (70 μM) over pH range from 3.0 to 7.0. (e) Values of fluorescence intensities of DCI2F-OTf treated with different competitive biological agents: 1. blank, 2. Na+ (500 μM), 3. K+ (500 μM), 4. Ser (200 μM), 5. Leu (200 μM), 6. Arg (200 μM), 7. S2O32- (100 μM), 8. HSO3- (100 μM), 9. H2S (100 μM), 10. Cys (100 μM), 11. GSH (1 mM), 12. NO2- (100 μM), 13. NO3- (100 μM), 14. NO (100 μM), 15. •OH (100 μM), 16. O21 (100 μM), 17. H2O2 (100 μM), 18. O2•- (100 μM), 19. HOCl (100 μM), 20. ONOO- (70 μM). (f) SDS-PAGE analysis of BSA (5 mg/mL) after incubation with DCI2F-OTf + ONOO-, DCI2F-OTf, DCI2F-OTf + ONOO- + UA in PBS at 37 ℃ for 30 min. Left: Coomassie brilliant blue staining; right: fluorescence imaging. λex/λem = 490/650 nm.
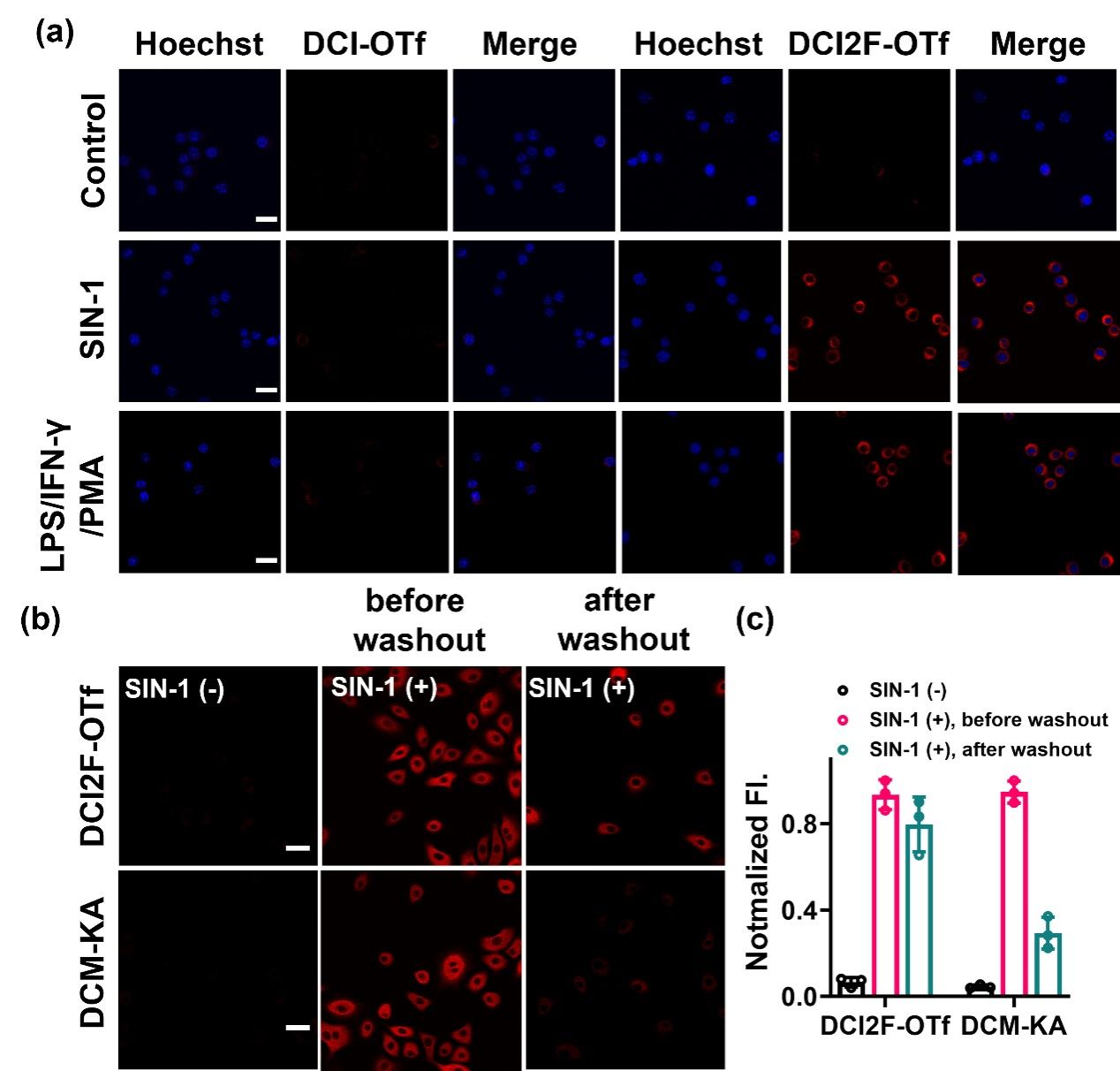
Figure 2. (a) RAW264.7 cells were pretreated with SIN-1 (200 μM, 30 min) or stimulated with LPS (1.0 μg/mL), IFN-γ (100 ng/mL) for 12 h, PMA (10 nM) for 30 min, and then incubated with DCI-OTf (10 μM, 30 min) or DCI2F-OTf (10 μM, 30 min), respectively. The red channel was collected in the range of 600-700 nm (λex = 488 nm) and the Hoechst channel was collected in the range of 430-480 nm (λex = 405 nm). (b) A549 cells were pretreated with SIN-1 (200 μM, 30 min), followed by incubation with DCI2F-OTf (10 μM, 30 min) or DCM-KA (10 μM, 30 min), before or after washout with fresh DMEM (three times, each for 6 min). (c) Normalized fluorescence intensity plots of DCI2F-OTf and DCM-KA labeled cells in (b) (n = 3). Scale bar: 50 μm.
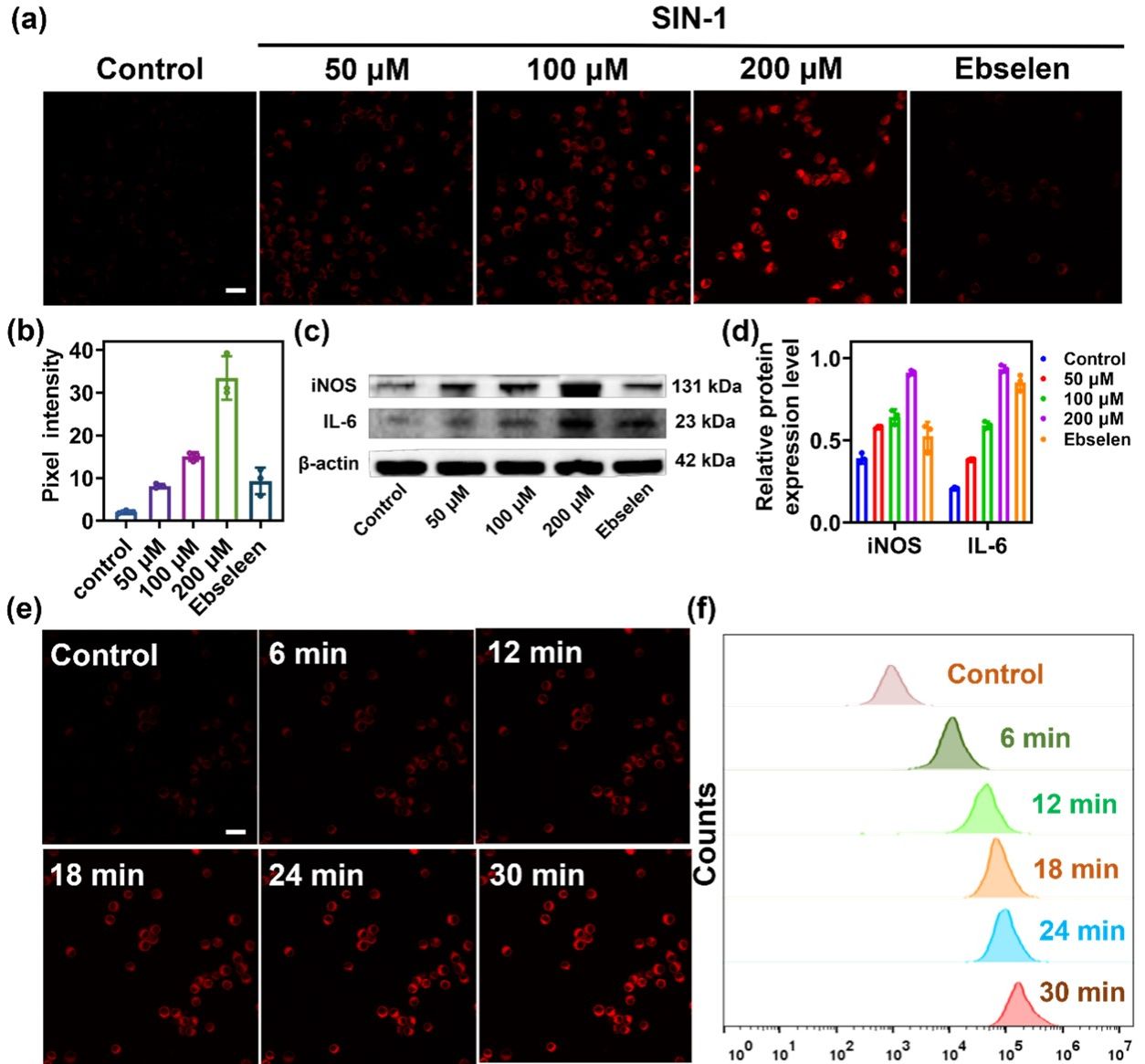
Figure 3. (a) RAW264.7 cells pretreated with different concentrations of SIN-1 (0, 50, 100, 200 μM), SIN-1 (200 μM) plus ebselen (200 μM) for 30 min were incubated with DCI2F-OTf (10 μM) for 30 min. (b) The pixel intensity of DCI2F-OTf labeled cells in (a). (c) The protein levels of iNOS and IL-6 were detected by western blot analysis. (d) The relative protein expression level of iNOS and IL-6 in (c). (e) RAW264.7 cells were pretreated with SIN-1 (200 μM, 30 min), and then incubated with DCI2F-OTf (10 μM) for 0 - 30 min. (f) Flow cytometry analysis of DCI2F-OTf (10 μM) labeled SIN-1 (200 μM, 30 min) pretreated cells. Error bars represent s.d., n=3. Scale bar: 50 μm.
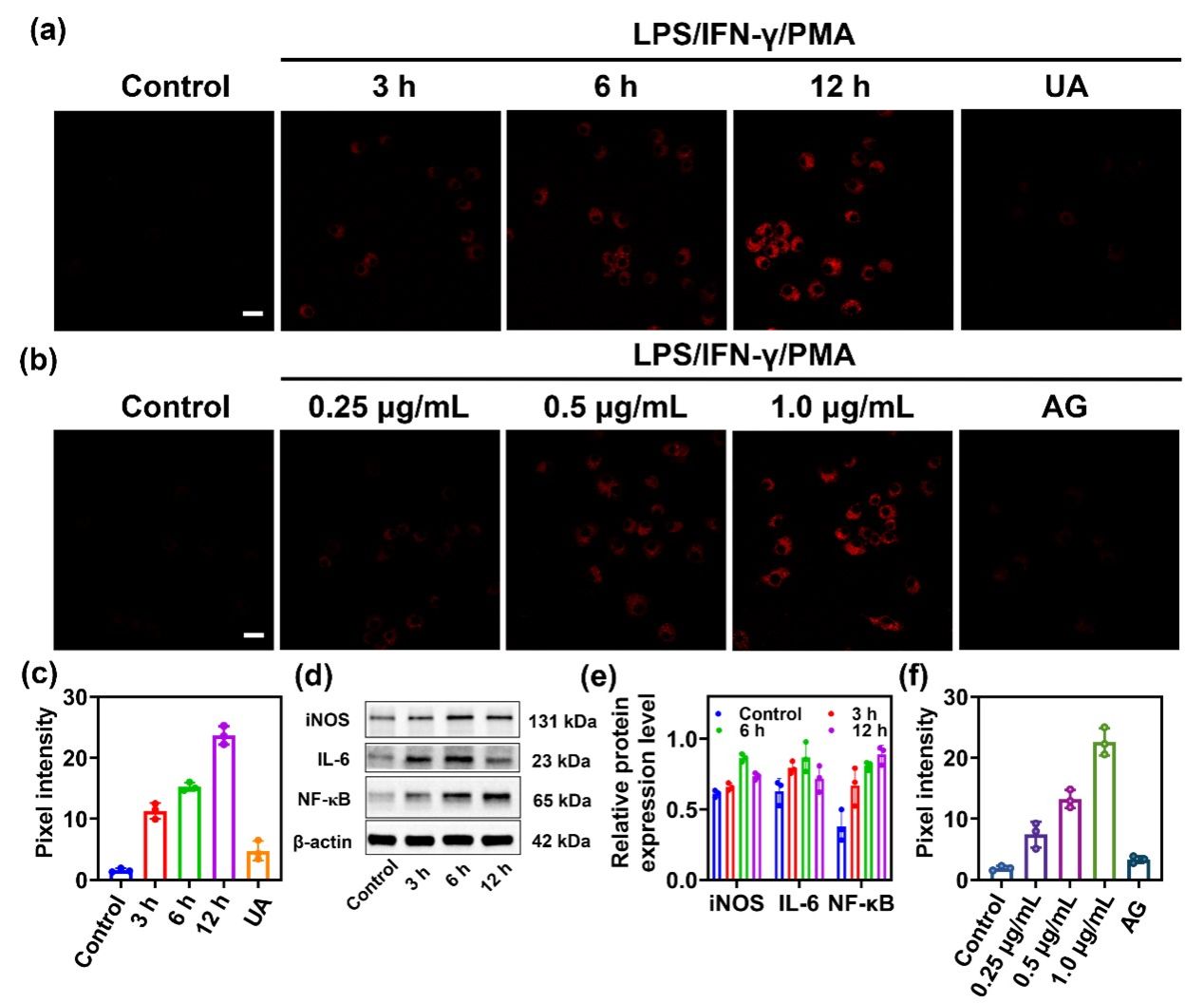
Figure 4. (a) RAW264.7 cells were pretreated with the stimulus LPS (1.0 μg/mL)/IFN-γ (100 ng/mL) for different periods (0, 3, 6, 12 h), PMA (10 nM) for 30 min or LPS (1.0 μg/mL)/IFN-γ (100 ng/mL) plus UA (200 μM) for 12 h, PMA (10 nM) for 30 min, and then incubated with DCI2F-OTf (10 μM) for 30 min. (b) RAW264.7 cells were pretreated with LPS (0, 0.25, 0.5, 1.0 μg/mL)/IFN-γ (100 ng/mL), PMA (10 nM), or LPS (1.0 μg/mL)/IFN-γ (100 ng/mL) plus AG (1 mM) for 12 h, PMA (10 nM) for 30 min, and then incubated with -OTf (10 μM) for 30 min. (c) The pixel intensity of DCI2F-OTf labeled cells in (a). (d) The protein levels of iNOS, IL-6, and NF-𝜅B were detected by western blot analysis. (e) The relative protein expression level of iNOS, IL-6, and NF-𝜅B in (c). (f) The pixel intensity of DCI2F-OTf labeled cells in (b). Error bars represent s.d., n=3. Scale bar: 50 μm.
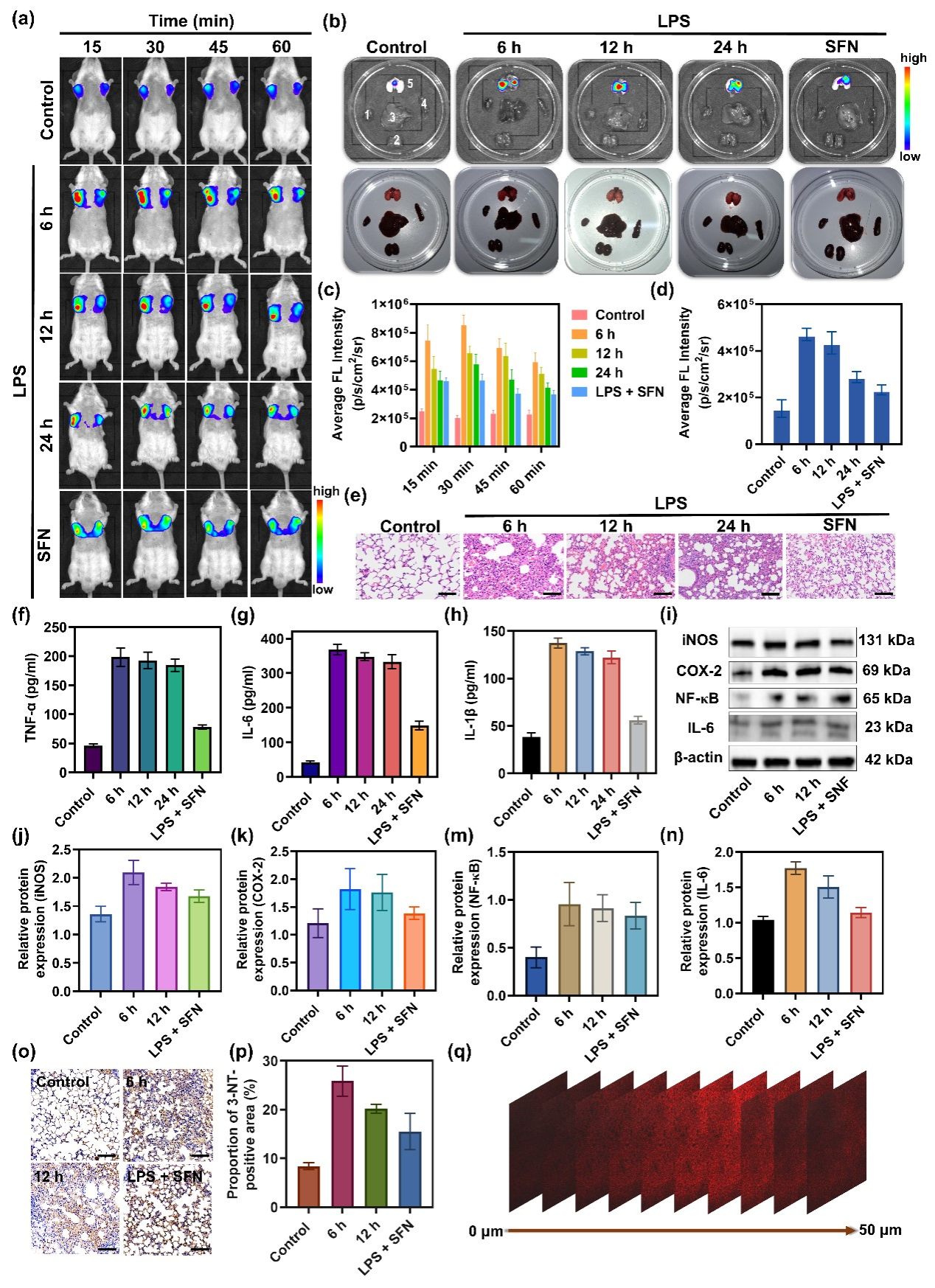
Figure 5. (a) NIRF images of control and LPS-stimulated mice after intravenous administration of DCI2F-OTf (200 μM, 45 μL). (b) NIRF imaging and photographs of dissected major organs (1: heart, 2: kidney, 3: liver, 4: spleen, 5: lung) from mice in panel (a). (c) The histogram presents the average radiant efficiency of mice in panel (a). (d) The histogram presents the average radiant efficiency of the lung in panel (b). (e) H&E staining histological images for lung tissue in control and LPS-stimulated mice. Scale bar: 50 μm. (f-h) Serum levels of inflammatory factors TNF-α, IL-6, and IL-1β in control and LPS-stimulated mice. (i) The protein levels of iNOS, COX-2, NF-κB, and IL-6 were detected by western blot analysis. (j-n) The relative protein expression level of iNOS, COX-2, NF-κB, and IL-6 in (i). (o-p) Sections of lung tissue were immunostained with an antibody that detected 3-NT. Scale bar: 50 μm. (q) NIRF images of mice fresh lung tissue sections from ALI mice in the LPS6 group. The confocal Z-axis scan images at 0, 5, 10, 15, 20, 25, 30, 35, 40, 45, and 50 µm penetration depths. λex = 488 nm; red channel: λem = 600 - 700 nm.
Conclusion
In conclusion, we have designed a novel NIRF probe, DCI2F-OTf, for fluorescence imaging ONOO- in live cells and mice model of ALI. Under physiological conditions, DCI2F-OTf was highly sensitive and selective for ONOO- and yielded a linear response to ONOO over a wide concentration range from 0 to 70 μM. The QM covalently labeled neighboring proteins released from the reaction of DCI2F-OTf with ONOO- could solve the problem of intracellular diffusion of the probe, enabling precise imaging of intracellular ONOO-. This strategy was superior to the vast majority of ONOO- probes that have been developed so far. The excellent sensing performance and NIRF emission characteristics enable DCI2F-OTf to image ONOO- levels in mice and tissues with ALI. In vivo imaging results indicated that SFN significantly down-regulated the oxidative stress level, thereby attenuating the severity of LPS-induced ALI, and could exert a protective role against ALI. Therefore, DCI2F-OTf was a valuable tool for studying the physiological function of ONOO- and was expected to facilitate the understanding of oxidative stress diseases such as ALI and the rapid screening of corresponding therapeutic drugs.