我院刘笑然/李景华团队联合mg4355线路检测官网第一附属医院在权威杂志《Acta Biomaterialia》上发表题为《Heterogeneous Cu2O-SnO2 Doped Polydopamine Fenton-like Nanoenzymes for Synergetic Photothermal-Chemodynamic Antibacterial Application》(中国科学院一区TOP期刊,IF=9.70)的研究论文。
研究者在前期相关研究基础上,深入研究铜基合金纳米材料在光激活光热-光动力-化学动力学活性抗菌/创面修复治疗中的应用。针对创口乏氧微环境,设计构筑了一种近红外光调控的基于自由基链式反应的自放大肿瘤靶向递送新策略体系,在Cu-Sn联合驱动类芬顿催化体系下在病灶部位生成过量活性氧,实现高效协同抗菌/创面修复效应,实现多模态影像协同介导下的可视化治疗,相关研究结果可为铜基复合合金材料在生物医学中的应用研究提供支撑。该研究得到国家自然科学基金(32260237)支持。
此系列研究推动了创面修复综合诊疗的技术创新,为相关疾病临床治疗提供参考。
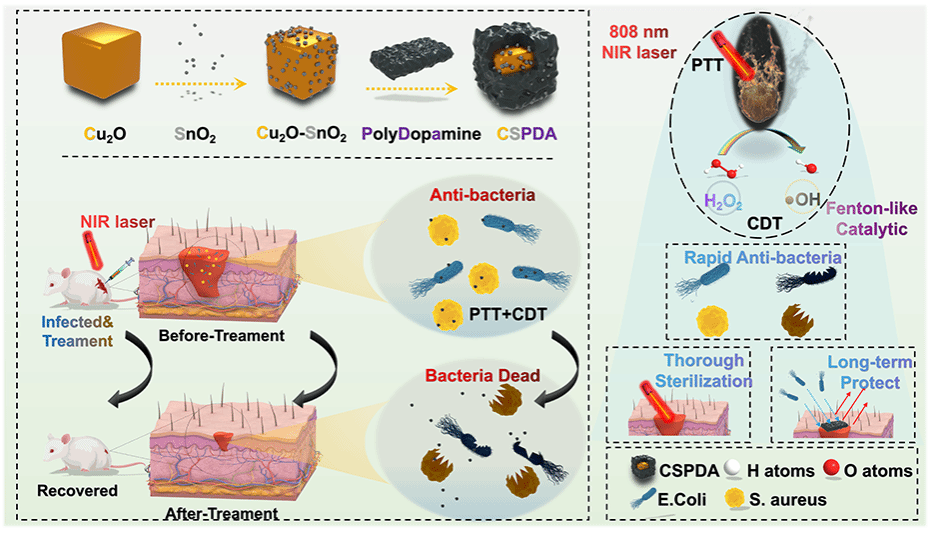
Heterogeneous Cu2O-SnO2 doped polydopamine fenton-like nanoenzymes for synergetic photothermal-chemodynamic antibacterial application
Author links open overlay panel,
,
,
,
,
,
,
,
,
- a
- The 1st Affiliated Hospital, College of Medical Technology and Engineering, Henan University of Science and Technology, Luoyang 471000, China
- b
- Key Laboratory of Hainan Trauma and Disaster Rescue, The 1st Affiliated Hospital, College of Emergency and Trauma, Hainan Medical University, Haikou 570100, China
- c
- Key Laboratory of Biorheological Science and Technology, Chongqing University, Chongqing 400044, China
- d
- Department of Radiation Oncology, The Second Department of Thoracic Surgery, The First Affiliated Hospital of Xi'an Jiaotong University, Xi'an, Shaanxi 710000, China
Received 17 August 2023, Revised 7 November 2023, Accepted 8 November 2023, Available online 17 November 2023, Version of Record 19 December 2023.
https://doi.org/10.1016/j.actbio.2023.11.009
Abstract
Wound infections caused by drug-resistant bacteria pose a great threat to human health, and the development of non-drug-resistant antibacterial approaches has become a research priority. In this study, we developed Cu2O-SnO2 doped polydopamine (CSPDA) triple cubic antibacterial nanoenzymes with high photothermal conversion efficiency and good Fenton-like catalase performance. CSPDA antibacterial nanoplatform can catalyze the generation of hydroxyl radical (·OH) from H2O2 at low concentration (50 μg∙mL−1) under 808 nm near-infrared (NIR) irradiation to achieve a combined photothermal therapy (PTT) and chemodynamic therapy (CDT). And the CSPDA antibacterial nanoplatform displays broad-spectrum and long-lasting antibacterial effects against both Gram-negative Escherichia coli (100 %) and Gram-positive Staphylococcus aureus (100 %) in vitro. Moreover, in a mouse wound model with mixed bacterial infection, the nanoplatform demonstrates a significant in vivo bactericidal effect while remaining good cytocompatible. To conclude, this study successfully develops an efficient and long-lasting bacterial infection treatment system. This system provided different options for future studies on the design of synergistic antimicrobial therapy. Hence, the as-synthesized synergetic photothermal therapy and chemodynamic therapy nanoenzymes have rapid and long-term bactericidal ability, well-conglutinant performance and effectively preventing wound infection for clinical application.

Scheme 1. Schematic diagram of CSPDA synthesis, flow of action, and functional profile of PTT and CDT synergistic antibacterial therapy.

Fig. 1. Characterization of the CSPDA. TEM image of (A) Cu2O, (B) Cu2O-SnO2 heterostructures, (C) CSPDA. (D) Distribution of elements: C, N, O, Cu and Sn on the CSPDA. (E) X-ray diffraction pattern of CSPDA. (F) XPS spectrum for CuSnO-DopaCube, (G) Cu 2p peak and (H) Sn 3d peak.

Fig. 2. Photothermal and catalytic capabilities of CSPDA. (A) Photothermal pictures and (B) temperature change curves of aqueous CSPDA solutions at different concentrations under NIR laser irradiation (808 nm and 1.4 W·cm−2·s − 1). (C) Temperature variation of CSPDA under different optical powers. (D) Temperature changes of CSPDA aqueous solutions (50 μg∙mL−1) during laser on/off cycling.(808 nm and 1.4 W·cm−2·s − 1). (E) Linear time data versus −lnθ obtained from the cooling period of laser off. (F) Color development of TMB by CSPDA+H2O2. (G) Concentration dependence of the TMB chromogenic reaction with H2O2. (H) Degradation effect of CSPDA+H2O2 on MB.

Fig. 3. In Vitro antibacterial activity of CSPDA. Photographs of colonies formed by (A) E. coli and (B) S.aureus treated with different concentrations of CSPDA with and without NIR. Antinbacterial rate for (C) E. coli and (D) S.aureus. (NIR:1.4 W·cm−2·s − 1, H2O2:1 mM).

Fig. 4. Photographs of the bacterial colonies formed by (A) S.aureus and (B) E.coli after the treated with PBS, H2O2, CSPDA, CSPDA+H2O2, (NIR: 1.4 W·cm−2·s-1, H2O2: 1 mM, CSPDA: 50 μg∙mL−1, Scale bars: 20 μm) and the corresponding cell live-dead staining (Viable bacteria are labeled green by calcein-AM and dead bacteria are labeled red by PI). (C) and (D) Survival rates corresponding to (A) and (B). (E) and (F) E. coli and S.aureus imaged by SEM after treatments (Scale bars: 2 μm).

Fig. 5. Long-term antibacterial activities of CSPDA at different concentration. (A) Optical photographs and (B) Relative pollution area after 14-day shallow immersion experiments with different concentrations of CSPDA.

Fig. 6. (A) Calculation and photo of hemolysis rate of different concentrations of CSPDA. (B) Cell activity of NIH-3T3 cells after incubation with different concentrations of CSPDA for 12 h. (C) Cell viability staining of NIH-3T3 cells after incubation with different concentrations of CSPDA for 12 (Scale bars: 20 μm).

Fig. 7. Antibacterial performance of Cu-GA in vivo. (A) Schematic illustrations of the experimental design and wound healing process. (B) Representative photographs of wound healing in different groups (Scale bars: 5 mm), and (C) the corresponding healing proportions. (D) Extraction of bacterial culture from different groups of wound locations after 7 days-treatment, and (E) relative bacterial viability. (F) Relative wound area over time in different groups.

Fig. 8. Pathological section of wound tissue. (A) H&E and (B) Masson staining of wound tissues: red rectangular box; hair follicles: green arrows; boundary of epithelium: black dotted lines). (Scale bars: 200 μm).
We have successfully developed a three-layered, multi-phase, heterogeneous nanoplatform based on Cu2O-SnO2-Polydopamine. In vitro experiments have confirmed that CSPDA exhibits biocompatibility and efficiently catalyzes the generation of hydroxyl radicals (·OH) from H2O2, thanks to its remarkable photothermal properties and Fen ton-like effects. This enables CSPDA to achieve long-lasting, rapid, and broad-spectrum synergistic sterilization through combined CDT and PTT. Furthermore, in vivo experiments and histological tests have demonstrated that CSPDA effectively eliminates bacteria, controls inflammation, promotes collagen deposition, and accelerates wound healing. Within 7 days, the wound healing rate exceeds 80 %. These findings highlight the immense potential of CSPDA in the treatment of wound infections. In summary, CSPDA represents a approach to address practical challenges such as drug-resistant bacteria and the overuse of antibiotics.